Currently, the most popular GLP1-based monotherapy, Semaglutide, controls blood sugar by promoting insulin secretion and induces a lasting sense of fullness to reduce appetite. However, this drug can cause side effects such as nausea and vomiting, which can affect patient adherence to the treatment. On July 10, 2024, Kuei-Pin Huang and colleagues published a paper in Nature titled “Dissociable Hindbrain GLP1R Circuits for Satiety and Aversion.” The study suggests that future weight loss medications could selectively target GLP1R neurons in the nucleus of the solitary tract (NTS) to avoid inducing aversion, thereby improving treatment adherence and effectiveness [1]. Today, let’s discuss GLP1R…
1. GLP1R Structure
In 1992, Thorens isolated and identified the first glucagon-like peptide-1 receptor (GLP1R) cDNA from rat pancreatic β-cells [2], and in the following year, in 1993, human GLP1R was cloned. Like the gastric inhibitory peptide receptor (GIPR), GLP1R belongs to the B1 class of G protein-coupled receptors (GPCRs). The human glucagon-like peptide-1 receptor (hGLP1R) is encoded by the GLP1R gene located on chromosome 6, spanning approximately 40 kb and containing around 14 exons. Both human and rat GLP1R have 463 amino acids, while mouse GLP1R has 489 amino acids, with homologies to hGLP-1R of 91% and 84%, respectively.
As with all B-class GPCRs, GLP1R features an N-terminal extracellular domain (ECD) consisting of 100-150 residues, connected to a complete 7-transmembrane (7TM) domain, which is a hallmark of all GPCRs. The 7TM domain comprises seven α-helices (TM1-TM7) separated by three intracellular loops (ICL1-ICL3) and three extracellular loops (ECL1-ECL3), with an additional intracellular C-terminal domain. The N-terminal ECD of the GLP1R protein can form a three-layer α-β-βα fold stabilized by three pairs of disulfide bonds between cysteine residues. This N-terminal ECD can bind to the C-terminal helix of glucagon-like peptide-1 (GLP-1), which contains key regions involved in ligand recognition and binding. Upon ligand binding, conformational changes occur in the N-terminal ECD, leading to the activation of intracellular signaling cascades. The transmembrane domain (TMD) binds to the N-terminal region of GLP-1 and contains a polar residue pivot that can modulate receptor signaling bias. The intracellular C-terminal domain interacts with intracellular signaling proteins, initiating downstream signaling pathways [4].
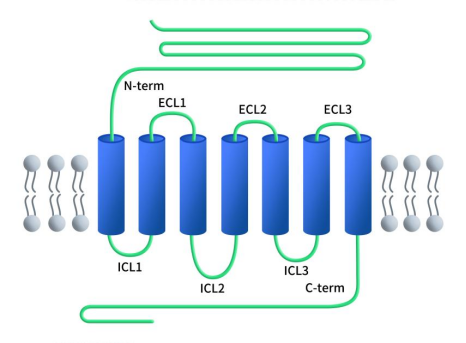
Figure 1. The structure of GLP1R [4]
2. Distribution and Function of GLP1R
GLP-1R is widely distributed across many tissues and organs, including the pancreas, stomach, duodenum, lungs, heart, kidneys, and brain. It is predominantly expressed in pancreatic β-cells. In the brain, the highest densities of GLP-1R are found in the nucleus of the solitary tract, posterior area, interpeduncular nucleus, posterior dorsal nucleus, ventral tegmental area, hypothalamic nuclei, thalamic nuclei, nucleus accumbens, and the lateral septum [5].
GLP1R exerts its biological functions by binding to GLP-1 and its analogs. In pancreatic β-cells, the primary role of GLP-1R is to promote β-cell proliferation, stimulate insulin synthesis and secretion, and inhibit glucagon synthesis and release. In the gastrointestinal tract and other tissues, GLP-1R can suppress gastric acid secretion and gastrointestinal motility, delay gastric emptying, enhance satiety, and reduce food intake. In neural tissues, GLP-1R signaling affects the central nervous system, particularly regions involved in appetite regulation. Activation of GLP-1R promotes a sense of fullness, which helps reduce food intake and improve weight management. In cardiovascular contexts, GLP-1R can enhance cardiovascular function and reduce inflammation [6].
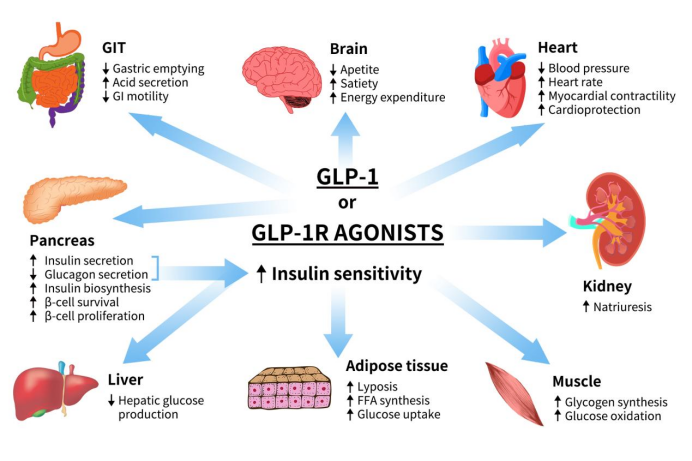
Figure 2. The role of GLP1 or GLP1R agonists [6]
3. Mechanism of GLP1R Signaling
As previously mentioned, GLP1R primarily exerts its biological functions by binding to its ligand GLP-1 and its analogs. This section focuses on the signaling mechanism of GLP1R in the pancreas. It is currently believed that GLP-1R mainly signals through the Gαs/cAMP pathway, although evidence also suggests that GLP-1R interacts with Gαq and other G proteins.
Upon activation of GLP-1R, the C-terminal undergoes phosphorylation, which further recruits β-arrestin, leading to receptor internalization and desensitization. The Gαs/cAMP pathway directly triggers glucose-induced insulin granule secretion. Once fully activated, GLP-1R couples with Gαs to activate adenylate cyclase (AC), converting ATP into cyclic adenosine monophosphate (cAMP), thereby increasing the concentration of cAMP. Elevated cAMP levels subsequently activate protein kinase A (PKA) and Rap guanine nucleotide exchange factor 4 (RAPGEF4, also known as EPAC2).
Activated PKA and EPAC2 can close ATP-dependent K+ channels and voltage-dependent K+ channels, leading to membrane depolarization and the activation of voltage-dependent calcium channels (VDCC), resulting in Ca2+ influx. In addition to the classical functions of cAMP, the cAMP/CREB pathway can induce the expression of insulin receptor substrate 2 (IRS2) and promote β-cell survival, further demonstrating the protective effects of GLP-1 analogs on β-cells [7] [8] [9] [10].
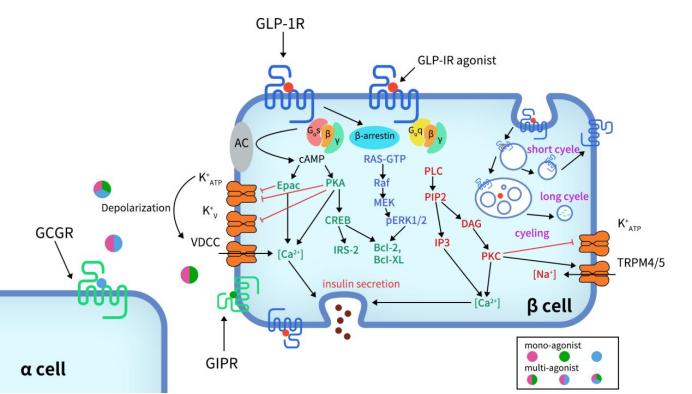
Figure 3. Signaling pathways of GLP-1R in pancreatic β-cell [11]
In addition to the Gαs/cAMP signaling pathway, GLP-1R can also couple with other G protein subtypes, such as Gαi, Gαq, Gαo, and Gα11. Recent studies have highlighted the significant role of the Gαq pathway in pancreatic β-cells. The Gαq pathway begins with the coupling to phospholipase C (PLC), which converts phosphatidylinositol 4,5-bisphosphate (PIP2) into inositol trisphosphate (IP3) and diacylglycerol (DAG). This process is accompanied by the activation of protein kinase C (PKC) and the production of intracellular Ca2+ influx through IP3 receptors. PKC also triggers the closure of ATP-dependent K+ channels and the activation of TRPM4/5 channels.
Gαs and Gαq regulate cAMP and Ca2+ levels [12] [13]. However, within the complex network of G protein signaling pathways, there are mechanisms of variation. Under certain conditions, such as sustained membrane depolarization and a reduction in GLP-1 concentration to picomolar levels, there can be a switch between Gαs and Gαq pathways [14]. This switching of G protein pathways elucidates a potential mechanism for basal insulin secretion under pharmacological treatment and provides an important direction for incretin-based therapies in type 2 diabetes.
4. Clinical Drug Research Progress for GLP1R
According to incomplete statistics, there are currently 413 GLP1R-targeting drugs worldwide, with nearly 110 in preclinical stages and a total of 154 in clinical stages or already on the market. Among these 154 drugs, 96 are single-target GLP1R drugs, while dual-target combinations include 19 for GIPR x GLP1R, 16 for GCGR x GLP1R, 7 for INSR x GLP1R, 3 for FGF21R x GLP1R, 2 for GLP-2R x GLP1R, and 1 for FGF21 x GLP1R. The types of drugs include synthetic peptides, recombinant proteins, small molecules, biosimilars, and fusion proteins. This overview focuses on single-target GLP1R drugs. For more information on the clinical research progress of dual-target GIPR x GLP1R drugs, please refer to [link or additional information].
- Approved and Marketed Drugs
Currently, 12 single-target GLP1R drugs have been approved for marketing, including 7 synthetic peptides and 5 recombinant peptides, all of which are GLP1R agonists. The earliest approved drug is Exenatide from Eli Lilly, launched in April 2005 and received orphan drug designation from the European Union. This was followed by Liraglutide from Novo Nordisk, approved in June 2009. Liraglutide is currently the top-selling GLP-1 receptor agonist globally, and between 2023 and 2024, five biosimilars of Liraglutide were approved. Lixisenatide from Zealand Pharma was approved in June 2013, targeting type 2 diabetes. In September 2014, Dulaglutide from Eli Lilly was approved and received priority review status in China. The highly popular weight loss drug Semaglutide, developed by Novo Nordisk, was launched in December 2017. It has received breakthrough therapy designation in China and, in the first half of 2024, ranked second in global sales among best-selling drugs, just behind Merck’s Keytruda.
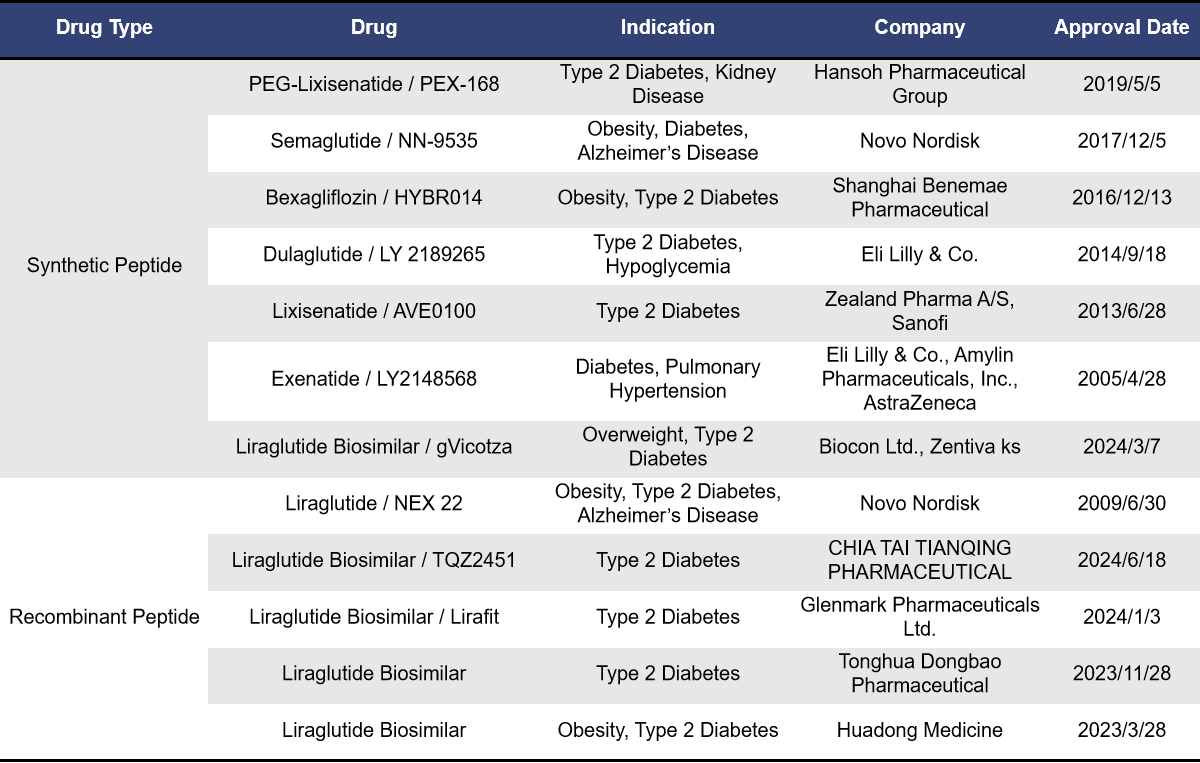
Liraglutide and Semaglutide are both GLP1R single-target agonists developed by Novo Nordisk. The development of GLP1R agonists primarily addresses the issue of the short half-life of natural GLP-1. Both Liraglutide and Semaglutide can suppress the appetite center, reducing human food intake by making people feel less hungry. They also delay gastric emptying, enhancing satiety and further reducing food consumption. Both drugs are administered via subcutaneous injection, but they differ in their duration of action. Liraglutide is a long-acting agonist, administered once daily, while Semaglutide is an ultra-long-acting agonist, administered once weekly.
- Drugs Under Market Approval Application
Currently, there are 8 single-target GLP1R drugs in the market approval application stage. These include synthetic peptides, fusion proteins, and recombinant peptides. Among them, 7 are GLP-1R agonists, including 3 biosimilars of Semaglutide.
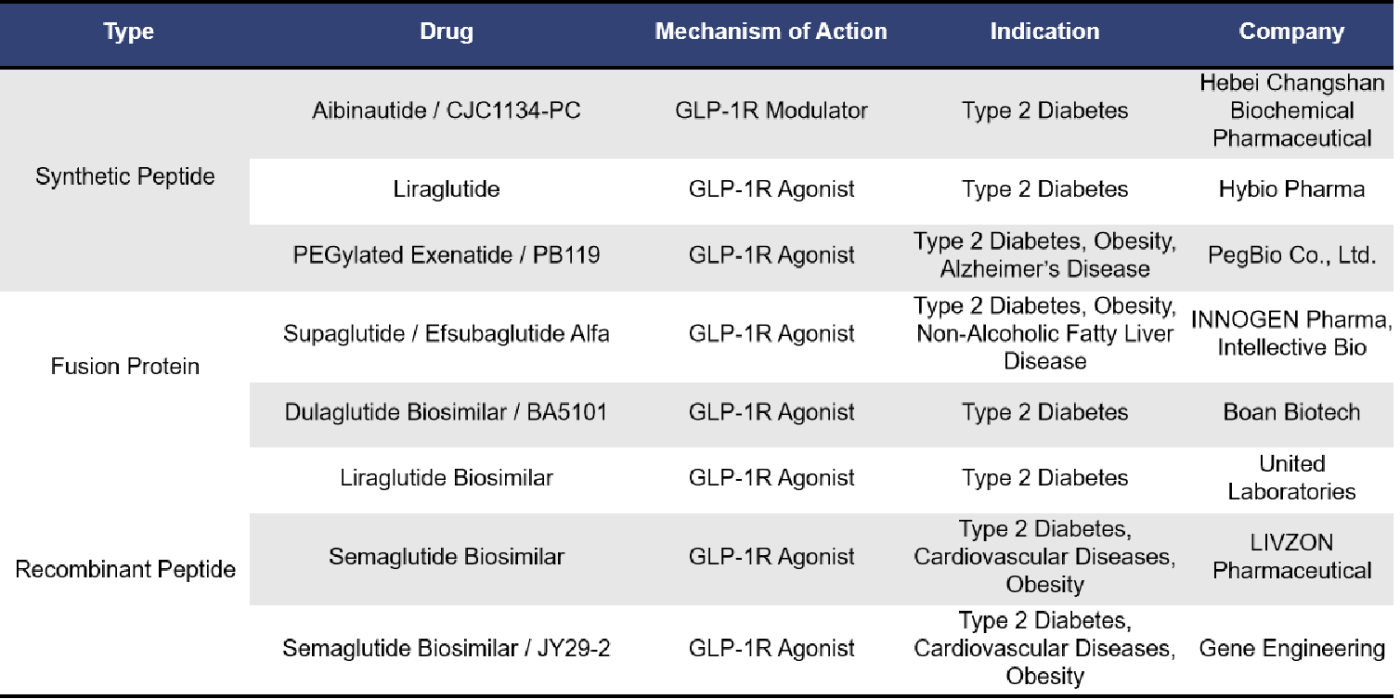
- Drugs In the Clinical Phase
There are 76 single-target GLP-1R drugs currently in clinical phase 1, 2 and 3 in the world, of which 26 are in clinical phase 3, 2 are in phase 2, 15 are in phase 2, 1 is in phase 1/2, and 32 are in phase 1. The types of drugs involved include synthetic peptides, small molecule drugs, recombinant peptides and fusion proteins, etc. Most of these drugs are mainly small molecule drugs and recombinant peptides.
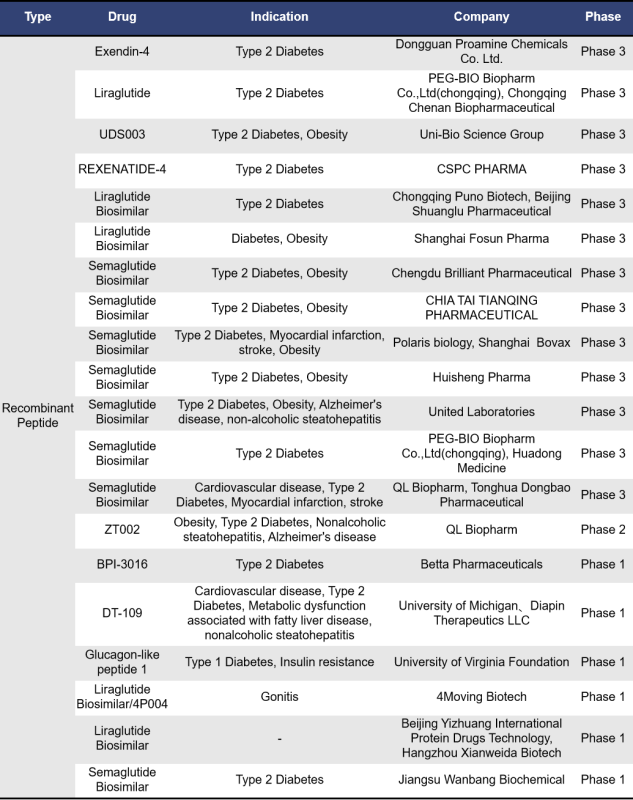
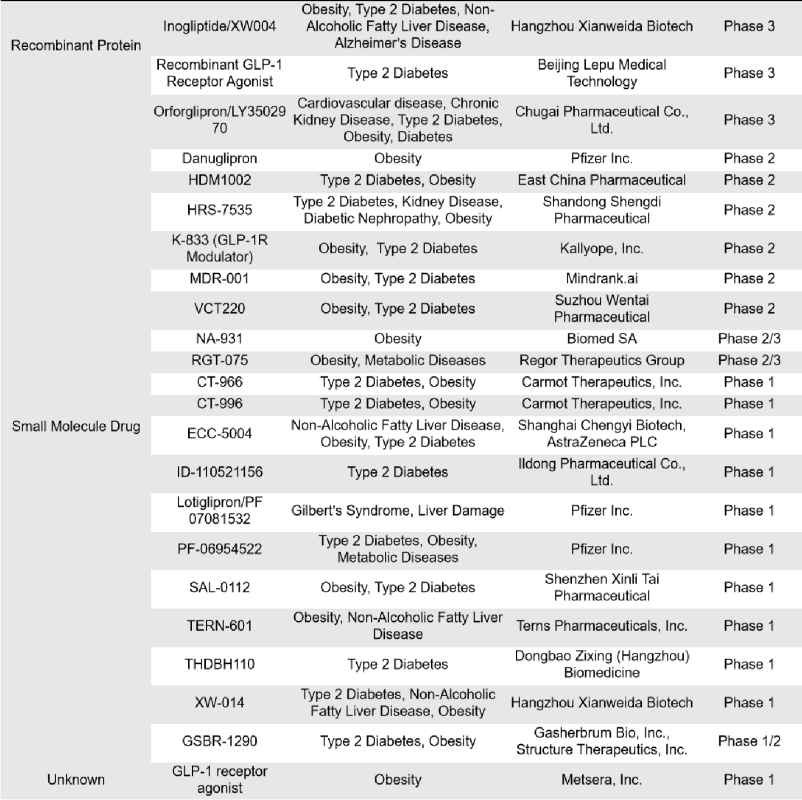
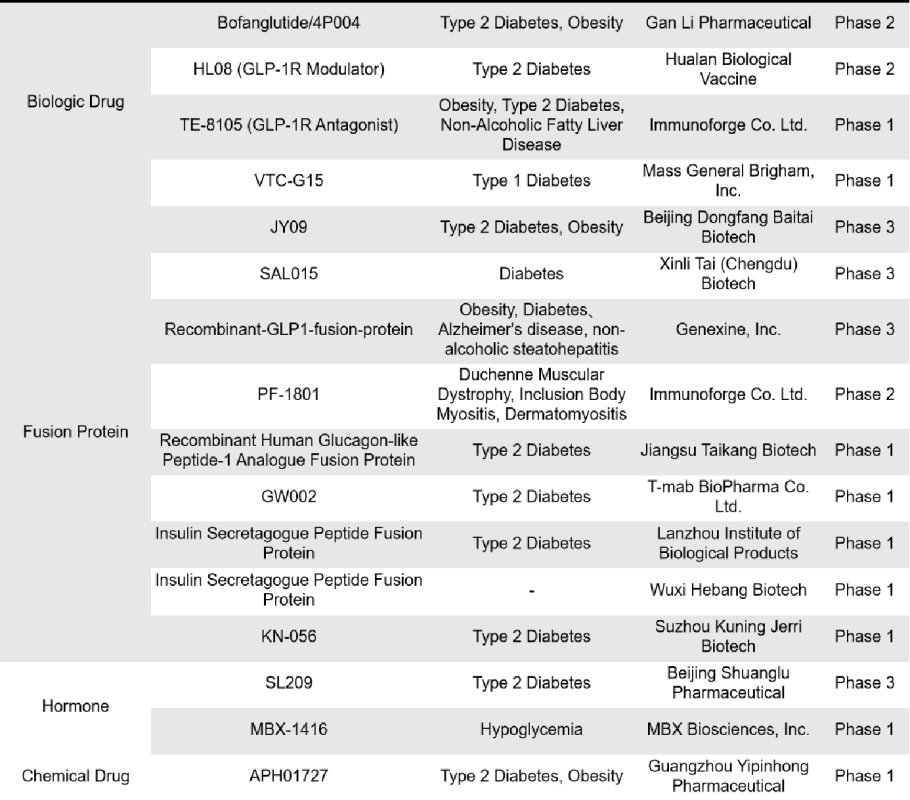
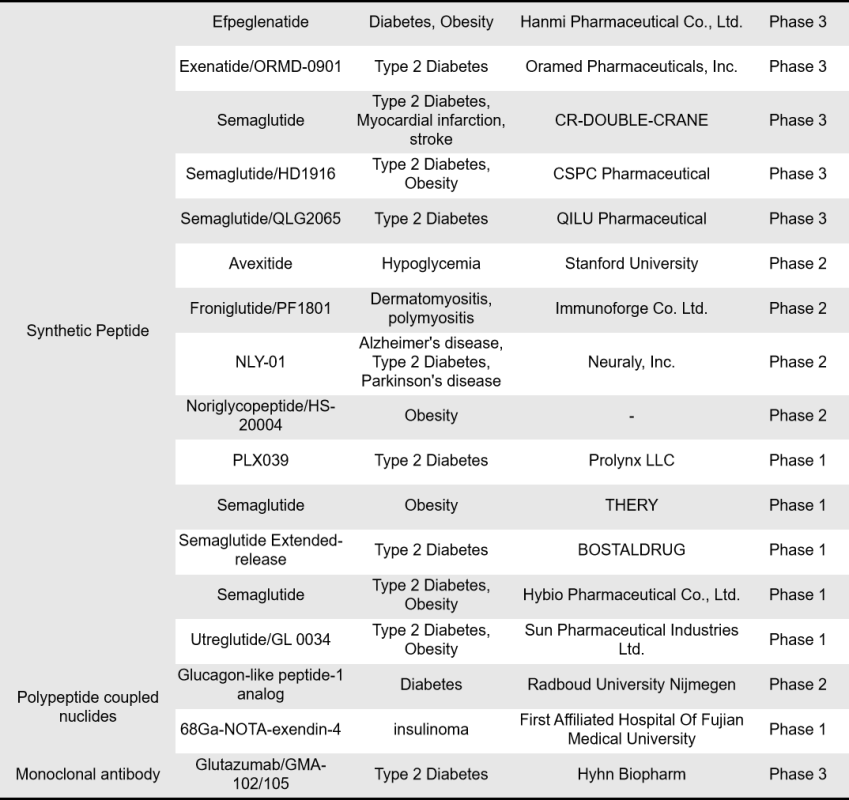
5. DIMA Biotechnology’s Synthetic Nanodisc Technology
DIMA Biotechnology is a biotechnology company focused on preclinical druggable target research and development products and services. Utilizing its proprietary Synthetic Nanodisc technology platform, DIMA Biotechnology has developed the full-length bioactive human GLP1R protein. Unlike most membrane scaffold protein (MSP) Nanodiscs available on the market, Dima Biotechnology’s Synthetic Nanodisc is developed using a eukaryotic expression system, enabling production directly from intact cells. The synthetic polymers used in this process serve two main purposes: they first dissolve the cell membrane, similar to a detergent, and then, using natural cellular phospholipids, they form a nanodisc structure around the membrane proteins. This allows the transmembrane proteins to be effectively integrated into the Nanodiscs.
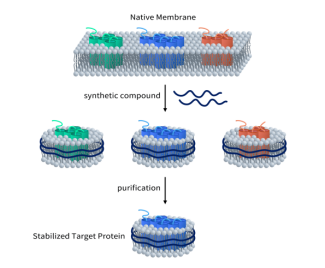
So far, DIMA has developed hundreds of full-length membrane proteins using their Synthetic Nanodiscs membrane protein platform. Click here to view all the membrane proteins.
Human GLP1R full-length protein-synthetic nanodisc (FLP100121)
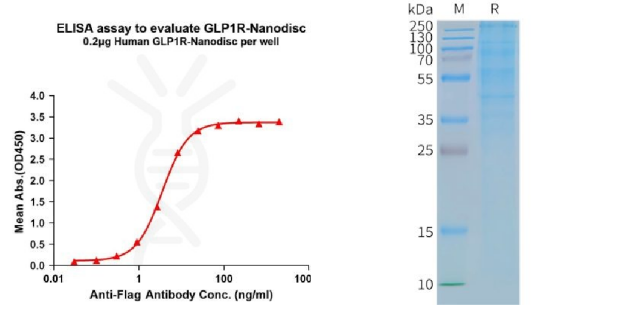
[left] Elisa plates were pre-coated with Flag Tag GLP1R-Nanodisc (0.2μg/per well). From above data, the EC50 for anti-Flag monoclonal antibody binding with GLP1R-Nanodisc is 3.549ng/mL. [Right] Human GLP1R-Nanodisc, Flag Tag on SDS-PAGE.
In addition, based on DIMA’s single B-cell antibody discovery, functional membrane protein development, antibody engineering modification, and functional validation platform, the company also provides a range of custom services. These include species-specific protein antibody customization, antibody humanization and affinity maturation, and lead molecule discovery services. For detailed information about these services, please feel free to contact us.
Reference:
[1]Huang, KP., Acosta, A.A., Ghidewon, M.Y. et al. Dissociable hindbrain GLP1R circuits for satiety and aversion. Nature (2024).
[2]Thorens B (1992) Expression cloning of the pancreatic beta cell receptor for the gluco-incretin hormone glucagon-like peptide 1. Proc Natl Acad Sci USA 89:8641–8645.
[3]Graaf, C.d, Donnelly, D., Wootten, D.,et al. Glucagon-Like Peptide-1 and Its Class B G Protein-Coupled Receptors: A Long March to Therapeutic Successes. Pharmacological reviews, 2016, 68(4), 954–1013.
[4]Donnelly D. The structure and function of the glucagon-like peptide-1 receptor and its ligands. British journal of pharmacology, 2012, 166(1), 27–41.
[5]Smith, N. K., Hackett, T. A., Galli, A., et al. GLP-1: Molecular mechanisms and outcomes of a complex signaling system. Neurochemistry international, 2019, 128, 94–105.
[6]Saraiva, F.K., Sposito, A.C. Cardiovascular effects of Glucagon-like peptide 1 (GLP-1) receptor agonists. Cardiovasc Diabetol, 2014, 13, 142.
[7]Marzook, A.; Tomas, A.; Jones, B. The Interplay of Glucagon-Like Peptide-1 Receptor Trafficking and Signalling in Pancreatic Beta Cells. Front. Endocrinol. 2021, 12, 678055.
[8]Kuna, R.S.; Girada, S.B.; Asalla, S., et al. Glucagon-like peptide-1 receptor-mediated endosomal cAMP generation promotes glucose-stimulated insulin secretion in pancreatic beta-cells. Am. J. Physiol. Endocrinol. Metab. 2013, 305, E161–E170.
[9]Lee, Y.S.; Jun, H.S. Anti-diabetic actions of glucagon-like peptide-1 on pancreatic beta-cells. Metabolism 2014, 63, 9–19.
[10]Rorsman, P.; Braun, M. Regulation of insulin secretion in human pancreatic islets. Annu. Rev. Physiol. 2013, 75, 155–179.
[11]Wan, W.; Qin, Q.; Xie, L.; et al. GLP-1R Signaling and Functional Molecules in Incretin Therapy. Molecules 2023, 28, 751.
[12]Bavec, A.; Hallbrink, M.; Langel, U.; et al. Different role of intracellular loops of glucagon-like peptide-1 receptor in G-protein coupling. Regul. Pept. 2003, 111, 137–144.
[13]Shigeto, M.; Ramracheya, R.; Tarasov, A.I.; et al. GLP-1 stimulates insulin secretion by PKC-dependent TRPM4 and TRPM5 activation. J. Clin. Investig. 2015, 125, 4714–4728.
Shigeto, M.; Cha, C.Y.; Rorsman, P.; et al. A role of PLC/PKC-dependent pathway in GLP-1-stimulated insulin secretion. J. Mol. Med. 2017, 95, 361–368.