Do you remember the temperature and touch receptor TRPV1 that made American scientists David Julius and Ardem Patapoutian accessible to the Nobel Prize in Physiology or Medicine in 2021? Transient Receptor Potential Vanilloid 1 (TRPV1) has gained significant attention in the field of pain treatment due to its potential as a therapeutic target. However, as research into TRPV1 has progressed, it has become increasingly clear that TRPV1 is not only associated with pain but also with other conditions such as inflammation, cardiovascular diseases, cough, mental disorders, and diabetes. Among these conditions, pain is the most extensively researched. Here, we will focus on summarizing the clinical research progress of TRPV1 in pain treatment. Before diving into this, let’s first review the biological background of TRPV1.
1. Structure of TRPV1
Based on amino acid sequence similarities, the Transient Receptor Potential (TRP) family can be divided into six subfamilies: TRPC (Canonical 1-7), TRPV (Vanilloid 1–6), TRPM (Melastatin 1–8), TRPA (Ankyrin 1), TRPP (Polycystic 1-3), TRPN (NOMP), and TRPML (Mucolopin 1-3) [1]. TRPV1 belongs to the vanilloid subfamily, which also includes TRPV2, TRPV3, TRPV4, TRPV5, and TRPV6. Among these, the TRPV subfamily is the most extensively studied. TRPV1 was the first mammalian TRP channel protein to be cloned from rat dorsal root ganglion (DRG) neurons in 1997 [2].
TRPV1 is also known as the capsaicin receptor, transient receptor potential cation channel subfamily V member 1, vanilloid receptor 1 (VR1), and Osm-9-like TRP channel 1 (OTRPC1). Human TRPV1 is located on chromosome 17p13.2 and consists of 19 exons encoding a protein of 838 amino acids with a molecular weight of 95 kDa. In 2008, Moiseenkova-Bell et al. first resolved the structure of the TRPV1 channel protein [3]. TRPV1 is a homotetramer with a fourfold symmetry around the central ion-conducting pathway. Each subunit has six transmembrane segments (S1-S6), with a reentrant loop (PL) between the fifth and sixth transmembrane helices (S5 and S6), forming a hydrophobic region. Both the N-terminus and C-terminus are located on the intracellular side of the membrane and are involved in receptor function regulation. The N-terminus contains multiple phosphorylation sites and six ankyrin repeat domains (note: some literature reports four repeat domains [4]), which can bind to calmodulin and ATP to modulate TRPV1 sensitivity and function [5]. The ankyrin repeat domains at the N-terminus consist of approximately 33-residue repeat units, with 24 such repeats connected by a 77-residue segment to S1 [6]. The C-terminus features a TRP domain, multiple calmodulin-binding domains, and binding sites for endogenous substances such as phosphatidylinositol 4,5-bisphosphate (PIP2) [7][8].
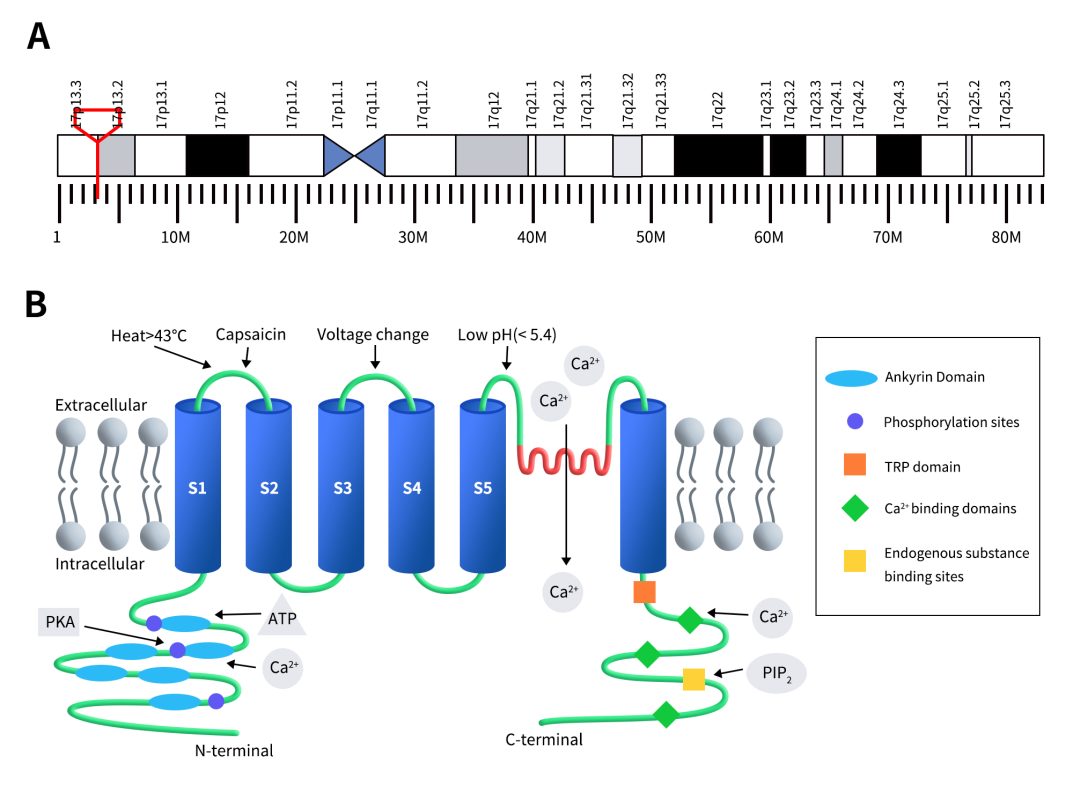
Figure 1. The gene location (A) and protein structure of TRPV1 (B) [5]
2. Distribution and Function of TRPV1
TRPV1 is predominantly expressed in the dorsal root ganglia (DRG), trigeminal ganglion (TG), and spinal cord [10]. It is also found in various regions of the central nervous system (CNS) such as the striatum, amygdala, thalamus, microglia, and astrocytes, as well as in non-neuronal tissues including hair follicles, mast cells, smooth muscle, keratinocytes, liver, tongue, oral cavity, bladder, kidneys, lungs, spleen, and cochlea. TRPV1 has been detected at low levels in regions such as the entorhinal cortex, olfactory bulb, hippocampus, and periaqueductal gray (PAG) [11]. Additionally, TRPV1 channels are widely distributed in various peripheral tissues and systems, including the vascular system, gastrointestinal tract, bladder, and immune system [12].
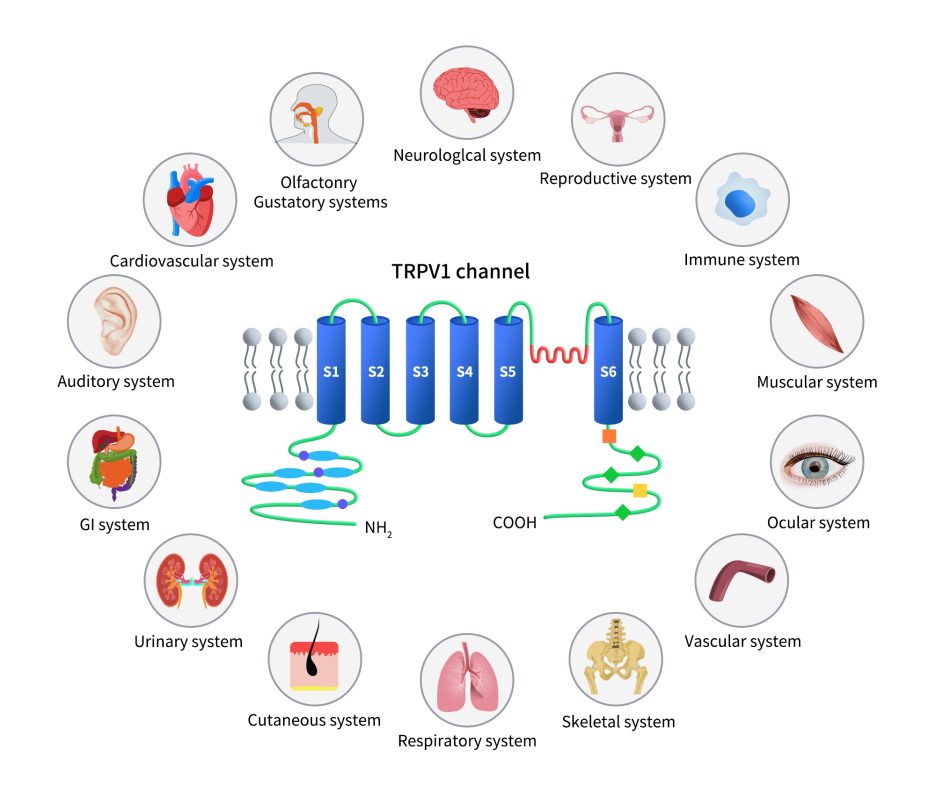
Figure 2. The distribution of TRPV1 expression [9]
Under normal conditions, TRPV1 is essential for various physiological processes. It helps in sensing temperature and oral sensations, regulates proteasome activity and autophagy, maintains energy balance, supports muscle function, controls gastrointestinal motility, and manages the release of inflammatory substances. It also plays a key role in the interaction between the immune system and sensory nerves.
Recent research has expanded our understanding of TRPV1’s functions. It is now known to affect insulin sensitivity, airway sensitivity, and bladder functions. A notable finding is its role in regulating immune responses: TRPV1 activation can influence macrophage behavior, leading to either inflammation or anti-inflammation depending on the situation. Additionally, TRPV1 has been linked to neurodegenerative diseases like Alzheimer’s and Parkinson’s, where it contributes to neuroinflammation.
3. The Mechanism of TRPV1 in Pain
TRPV1, due to its involvement in various physiological processes, is linked to a wide range of diseases, including temperature regulation disorders, pain, inflammation, cancer, neurological disorders, auditory and visual impairments, loss of smell or taste, infections, reproductive system disorders, respiratory conditions, obesity, cardiovascular diseases, diabetes, skin disorders, urinary tract diseases, muscle disorders, and skeletal system diseases [13]. Among these, the research on TRPV1’s role in pain and inflammation is particularly extensive.
As illustrated in the diagram below, TRPV1 is associated with numerous intracellular signaling pathways that regulate channel activity, especially in pain and inflammation. Various G protein-coupled receptors (GPCRs) are activated by pro-inflammatory agents such as histamine and prostaglandins. Activation of Gs protein-coupled receptors leads to the activation of adenylate cyclase (AC), which produces cAMP and activates protein kinase A (PKA). The activated PKA then directly regulates TRPV1 activity by phosphorylation.
Similarly, Gq protein-coupled receptor activation results in the activation of phospholipase C (PLC), which breaks down membrane-associated PIP2 into diacylglycerol (DAG) and inositol trisphosphate (IP3). Increased IP3 levels cause the release of Ca2+ from intracellular stores such as the endoplasmic reticulum (ER). Both Ca2+ and DAG activate protein kinase C (PKC), which also phosphorylates TRPV1 and modulates its function. PIP2 can have either positive or negative effects on TRPV1 channel regulation, depending on the receptor’s status.
Extracellular signals such as nerve growth factor (NGF) activate TRK receptors, which can also trigger PLC activation. The activation of TRPV1 or voltage-dependent calcium channels (VDCCs), or the release of intracellular calcium stores induced by pro-inflammatory factors, leads to increased intracellular Ca2+. This rise in Ca2+ modulates TRPV1 function by binding to calmodulin associated with TRPV1 and promoting channel desensitization. Calcium-calmodulin-dependent kinase II (CaMKII) directly phosphorylates the TRPV1 channel. Mechanical stimulation activates integrin-dependent src protein tyrosine kinase (srcPTK), which directly affects TRPV1. Additionally, voltage-dependent sodium or calcium (VDSC) channel activation causes cellular depolarization that directly gates the TRPV1 channel.
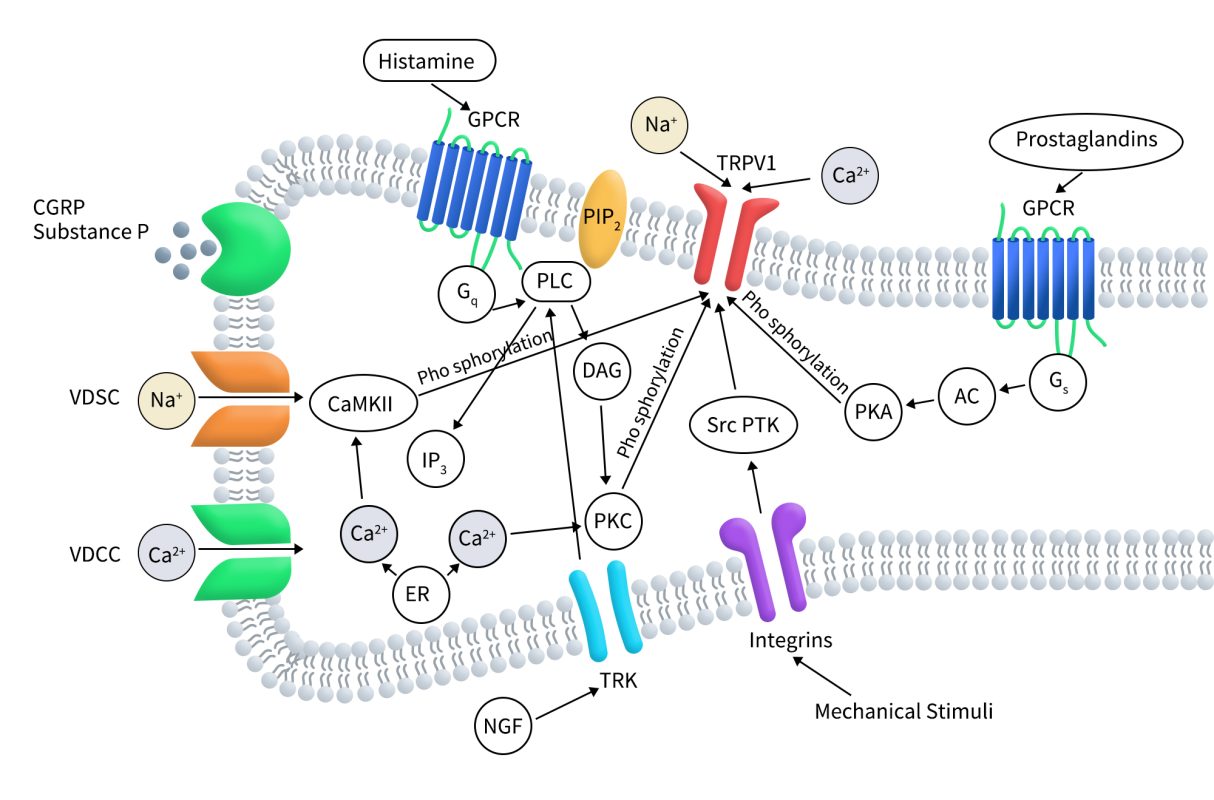
Figure 3. TRPV1 regulation by intracellular signaling pathways [14]
4. Clinical Research Progress of TRPV1-Targeted Drugs
Globally, there are currently 106 TRPV1-targeted drugs under investigation. Of these, 31 are in preclinical stages, 49 have been terminated or show no progress, and 3 have been approved and are on the market. The remaining 15 drugs are in various phases of clinical trials. These drugs encompass several types, including small molecules, synthetic peptides, and siRNA, with small molecules being the most prevalent, totaling nearly 89. Notably, all three approved drugs are small molecules. Below is a summary of the TRPV1-targeted drugs that are currently in clinical development.
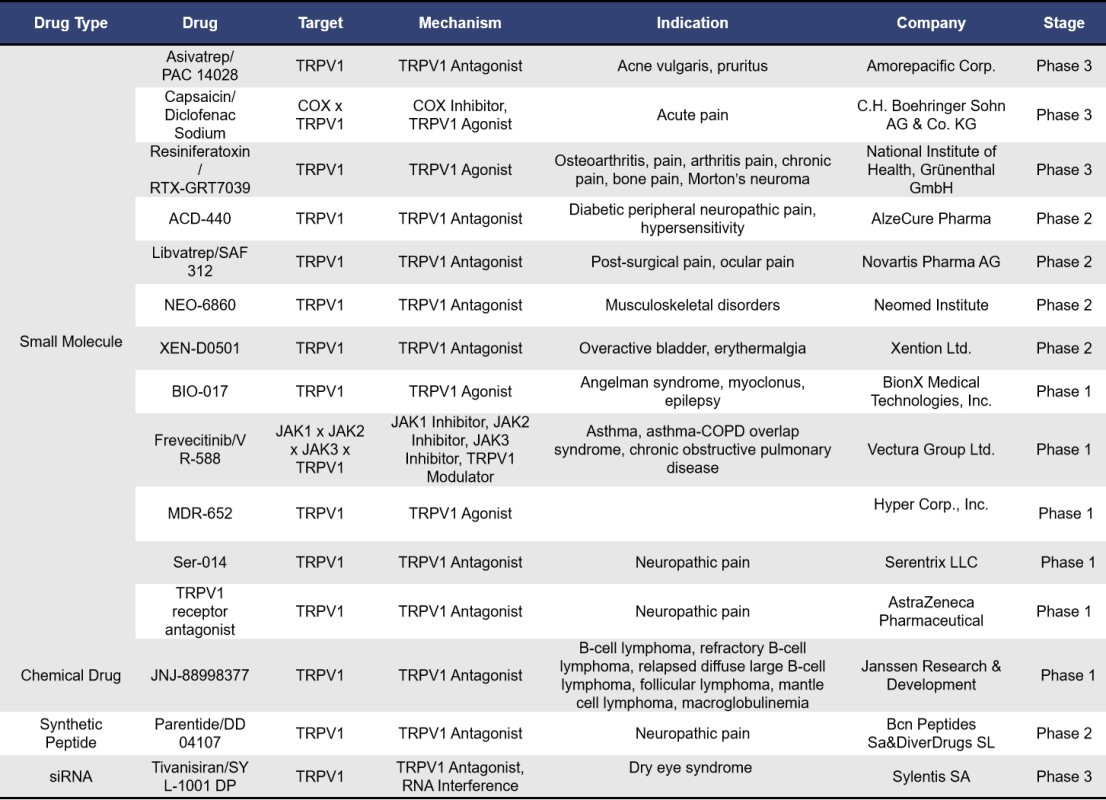
Among the 15 TRPV1-targeted drugs currently in clinical stages, 12 are small molecules, 1 is a synthetic peptide, 1 is a siRNA, and 1 is a chemical drug.
Asivatrep, also known as PAC14028, is a TRPV1 antagonist developed by Amorepacific Corp. It exerts anti-pruritic effects by inhibiting the release of neuropeptides such as substance P and by improving the skin barrier, potentially playing a significant role in the management of itching and inflammation in atopic dermatitis (AD).
A multicenter, randomized, double-blind Phase IIb clinical trial (NCT02757729) included 194 adult patients with mild to moderate AD. Participants were treated with 0.1%, 0.3%, or 1.0% PAC14028 cream, or a placebo, applied topically for 8 weeks. The results showed an overall response rate (IGA) of 42.55% for the 0.1% dose, 38.30% for the 0.3% dose, 57.45% for the 1.0% dose, and 14.58% for the placebo, with significant statistical differences.
Currently, a Phase III clinical trial (NCT02965118) is underway to evaluate the efficacy and safety of PAC14028 in treating adolescent patients with AD.
RTX-GRT7039, also known as MTX-071, is a TRPV1 agonist. Resiniferatoxin (RTX) binds to TRPV1, prolonging the channel’s open time and significantly increasing calcium ion influx, which leads to cytotoxic effects on TRPV1-expressing sensory neurons. Currently, Grünenthal GmbH is advancing three Phase III clinical trials (NCT05248386, NCT05449132, and NCT05377489) for RTX-GRT7039, all targeting osteoarthritis. These trials were initiated in August 2022 and are yet to begin recruitment.
ACD-440, developed by AlzeCure Pharma, is a TRPV1 antagonist designed for topical treatment of peripheral neuropathic pain. The drug completed a positive Phase Ib study in 2021, demonstrating excellent tolerance and safety. Applied as a gel on the skin, ACD-440 has shown effective and long-lasting analgesic effects. In 2023, a Phase II clinical trial (NCT05416931) was completed. This double-blind, placebo-controlled, randomized crossover study aimed to evaluate the efficacy, safety, and pharmacokinetics of ACD-440. The results of this trial have not yet been released.
Parentide, also known as DD 04107 or DD-04107, is a TRPV1 antagonist developed by Bcn Peptides SA and DiverDrugs SL. This synthetic peptide drug is currently in Phase II clinical development for the treatment of neuropathic pain.
Tivanisiran, also known as SYL1001 DP, is a siRNA drug targeting TRPV1 developed by Sylentis for the treatment of dry eye disease. Administered as an eye drop solution, Tivanisiran is currently in Phase III clinical trials. These trials evaluate the safety and efficacy of Tivanisiran for treating dry eye disease and dry eye syndrome caused by Sjögren’s syndrome (NCT05310422; NCT04819269). Both clinical trials have been completed, but the results have not yet been published.
5. Full-Length TRPV1 Protein by DIMA Biotech: Advancing New Drug Development
As previously mentioned, TRPV1 is a channel protein with six transmembrane domains. Whether for antibody drugs or small molecules, obtaining an active full-length membrane protein is crucial. DIMA Biotech has developed a full-length human TRPV1 protein using its proprietary Synthetic Nanodisc membrane protein expression technology platform.
Unlike most membrane scaffold protein (MSP) Nanodiscs available on the market, DIMA’s Synthetic Nanodisc is based on an eukaryotic expression system, allowing for direct productionof the full length membrane proteins from intact cells. In this process, the synthetic polymer used serves a dual purpose. First, it dissolves the cell membrane, similar to a detergent. Then, it uses natural cell phospholipids to form a nanodisc structure around the membrane protein. The transmembrane proteins can be integrated into these Nanodiscs.
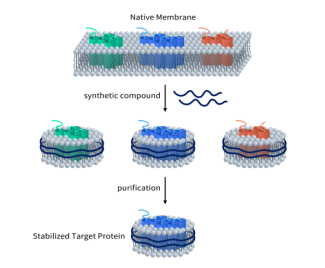
Currently, DIMA has developed hundreds of membrane proteins using its Nanodiscs full-length membrane protein platform. Click here to view all the membrane proteins.
Human TRPV1 full-length protein-synthetic nanodisc (FLP100128)
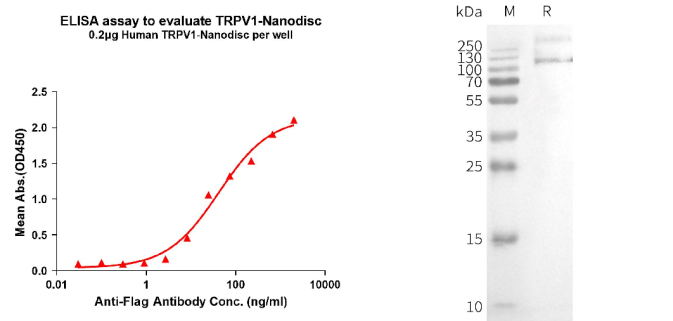
[left] Elisa plates were pre-coated with Flag Tag TRPV1-Nanodisc (0.2μg/per well). From above data, the EC50 for anti-Flag monoclonal antibody binding with TRPV1-Nanodisc is 41.79ng/ml. [Right] WB analysis of Human TRPV1-Nanodisc with anti-Flag monoclonal antibody.
References:
[1] Talavera K., Startek J. B., Alvarez-Collazo J., et al. Mammalian transient receptor potential TRPA1 channels: From structure to disease [J]. Physiol. Rev. 2020,100, 725–803.
[2] Caterina MJ, Schumacher MA, Tominaga M, et al. The capsaicin receptor: a heat-activated ion channel in the pain pathway. Nature. 1997 Oct 23;389(6653):816-24.
[3] Moiseenkova-Bell, V. Y., Stanciu, L. A., Serysheva, I. I., et al. Structure of TRPV1 channel revealed by electron cryomicroscopy. Proceedings of the National Academy of Sciences of the United States of America, 2008, 105(21), 7451–7455.
[4] Ramal-Sanchez, M., Bernabò, N., Valbonetti, L., et al. Role and Modulation of TRPV1 in Mammalian Spermatozoa: An Updated Review. International journal of molecular sciences, 2021, 22(9), 4306.
[5] Xiao T, Sun M, Kang J, Zhao C. Transient Receptor Potential Vanilloid1 (TRPV1) Channel Opens Sesame of T Cell Responses and T Cell-Mediated Inflammatory Diseases. Front Immunol. 2022 May 11;13:870952.
[6] Ma, L., Yang, F., Vu, S., & Zheng, J.. Exploring functional roles of TRPV1 intracellular domains with unstructured peptide-insertion screening. Scientific reports, 2016, 6, 33827.
[7] Garcia-Sanz N, Fernandez-Carvajal A, Morenilla-Palao C, et al. Identification of a Tetramerization Domain in the C Terminus of the Vanilloid Receptor. J Neurosci, 2004, 24(23):5307–14.
[8] Numazaki M TT, Takeuchi K, Murayama N, et al. Structural Determinant of TRPV1 Desensitization Interacts With Calmodulin. Proc Natl Acad Sci USA, 2003, 100(13):8002–6.
[9] Jaffal SM. Role of TRPV1 in Health and Disease. J Explor Res Pharmacol. 2023;8(4):348-361.
[10] Caterina MJ, Schumacher MA, Tominaga M, et al. The capsaicin receptor: a heat-activated ion channel in the pain pathway. Nature 1997;389(6653):816-824.
[11] Cavanaugh DJ, Chesler AT, Jackson AC, et al. Trpv1 reporter mice reveal highly restricted brain distribution and functional expression in arteriolar smooth muscle cells. J Neurosci 2011;31(13):5067-5077.
[12] Li YR, Gupta P. Immune aspects of the bi-directional neuroimmune facilitator TRPV1. Mol Biol Rep 2019;46(1):1499-1510.
[13] Jaffal SM. Role of TRPV1 in Health and Disease. J Explor Res Pharmacol. 2023;8(4):348-361.
[14] Jara-Oseguera A, Simon SA, Rosenbaum T. TRPV1: on the road to pain relief. Curr Mol Pharmacol. 2008 Nov;1(3):255-69.