Antibody-drug conjugates (ADCs) represent a promising class of targeted therapies in the fight against cancer. These innovative therapies aim to deliver small-molecule chemotherapy agents directly to cancer cells while sparing healthy ones. Since the approval of MYLOTARG in 2000, ADCs have become a vital category in the realm of cancer-targeted treatments, consisting of three key components: monoclonal antibodies, linkers, and toxins. Monoclonal antibodies play a pivotal role in determining the efficacy of ADCs, linkers influence their systemic toxicity and clinical performance, and toxins serve as the critical factors in determining the killing power of these drugs. Although there are over 130 different toxins reported in ADCs, only 6-8 toxins are used in ADCs currently in clinical trials or on the market. These toxins can be broadly categorized into three types: microtubule inhibitors, DNA synthesis inhibitors, and topoisomerase inhibitors. Among these, MMAE, a microtubule inhibitor, has achieved the most approvals as an ADC toxin. In this article, we will delve into the definition of MMAE and its applications and mechanisms within ADCs.
1. The Evolution of Toxins in ADCs
3. MMAE’s Application and Mechanism in ADCs
4. How Anti-MMAE Antibodies Facilitate ADC Drug Development
1. The Evolution of Toxins in ADCs
Before we introduce MMAE, let’s take a brief look at the history of toxins used in ADCs. The concept of ADCs was first proposed by Nobel laureate Paul Ehrlich in 1913. However, the development of ADCs began in 1975 when hybridoma technology was first used for monoclonal antibody production. Over almost five decades of research and development, ADCs have undergone three major iterations. Each generation of ADCs employed toxins with unique characteristics:
First-generation ADCs predominantly used traditional chemotherapy agents such as calicheamicin as toxins. However, these toxins had limited cytotoxicity against cancer cells, lacked specificity, and couldn’t accumulate effectively within target cells. Consequently, the first-generation ADCs often had lower efficacy than standalone chemotherapy drugs. They also suffered from issues like unstable linkers, highly hydrophobic toxins, and subpar conjugation techniques.
Second-generation ADCs underwent a comprehensive optimization of all three key components: antibodies, linkers, and toxins. In the realm of toxins, second-generation ADCs mainly employed microtubule inhibitors, including MMAE and MMAF. These novel highly cytotoxic compounds were 100 to 1000 times more potent against cancer cells than the traditional chemotherapy drugs used in the first-generation ADCs. However, these highly potent toxins, when used as standalone drugs for killing tumors, often produced intolerable side effects, which prevented their approval for standalone cancer treatment. Nevertheless, they proved to be ideal candidates for ADC development due to their heightened effectiveness against rapidly dividing tumor cells. One drawback was their preference for dividing tumor cells over static cancer cells.
Third-generation ADCs sought to overcome this limitation by selecting DNA-damaging agents capable of targeting the entire cell cycle. These toxins included DNA-damaging agents like enediynes, topoisomerase I inhibitors, and pyrrolobenzodiazepines (PBDs). DNA-damaging agents kill tumor cells through mechanisms involving double-strand breaks, alkylation, intercalation, and cross-linking of DNA strands [1]. In addition to advances in conjugation techniques and antibody selection, third-generation ADCs addressed the issues of their predecessors.
Despite three iterations of ADC development, the clinical application of ADC toxins still has limitations such as severe side effects and drug resistance. Among the 15 approved ADC drugs, MMAE serves as the toxin in five of them.
2. What Is MMAE?
Monomethyl auristatin E (MMAE) is a cytotoxic agent capable of killing cancer cells. MMAE is a derivative of dolastatin 10, a natural compound found in the Indian Ocean sea hare (Dolabella auricularia). Dolastatin 10, a microtubule inhibitor, exerts significant cytotoxicity and has been extensively used as a payload component in ADCs. MMAE and another derivative, monomethyl auristatin F (MMAF), are commonly used toxins in ADCs. MMAE consists of four amino acids: monomethylvaline (MeVal), valine, dolaisoleucine, and dolaproine, along with a C-terminal amine-bearing auristatin. In MMAF, the C-terminus of monomethylvaline is substituted with phenylalanine, significantly reducing its cellular activity. MMAE, in essence, is a demethylated form of auristatin E, with only one methyl substituent on the N-terminal amine, as opposed to auristatin E, which has two [2]. MMAE is a highly potent synthetic anticancer agent, exhibiting 100 to 1000 times more potency than auristatin E. Due to its toxicity, MMAE cannot be used as a standalone drug. However, when coupled with monoclonal antibodies, it can be directed to specific cancer cells due to the antibodies’ ability to recognize specific markers on cancer cells.
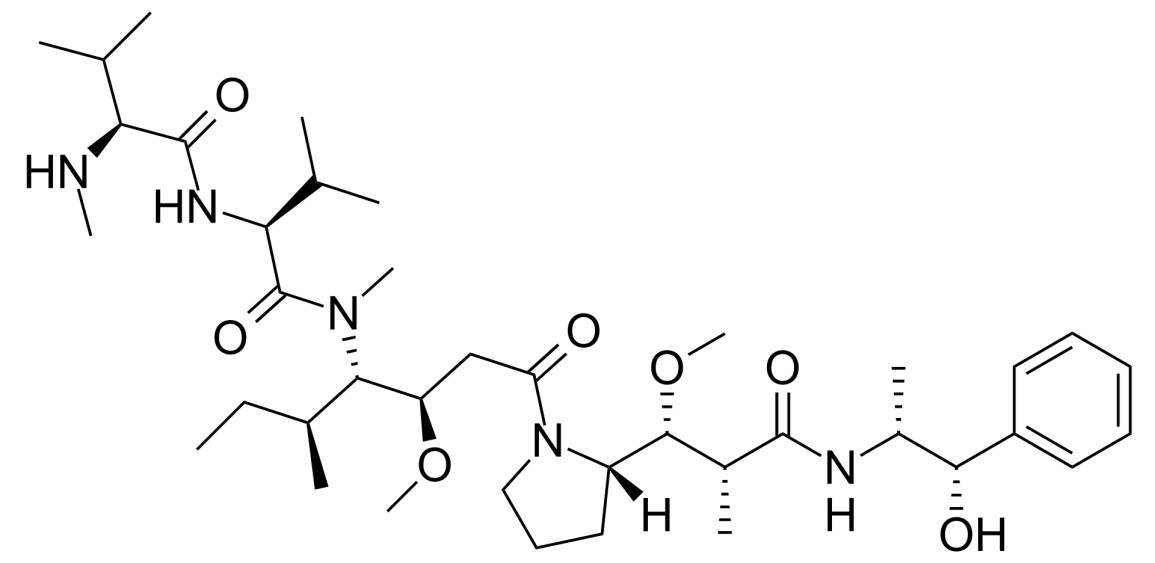
Figure 1. the structure of MMAE (Origin from Wikipedia)
3. MMAE’s Application and Mechanism in ADCs
As previously mentioned, MMAE, a widely used small molecule drug, is applied extensively in the development of antibody-drug conjugates (ADCs) for the treatment of various cancer types. It is currently one of the most widely used effective payloads in the ADC field. To illustrate MMAE’s mechanism of action, we will focus on Adcetris, an ADC that employs MMAE.
Adcetris, also known as brentuximab vedotin, is an ADC targeting CD30, co-developed by Takeda Pharmaceuticals in Japan and Seattle Genetics in the United States. Adcetris received FDA approval in 2011 for the treatment of conditions such as systemic anaplastic large cell lymphoma (sALCL), classical Hodgkin lymphoma (cHL), primary cutaneous anaplastic large cell lymphoma (pcALCL), or mycosis fungoides (MF). Adcetris consists of three components: an antibody targeting human CD30, a linker that can be proteolytically cleaved, and MMAE. Each antibody molecule carries approximately four MMAE molecules, allowing Adcetris to deliver high concentrations of MMAE within target cells for therapeutic effect.
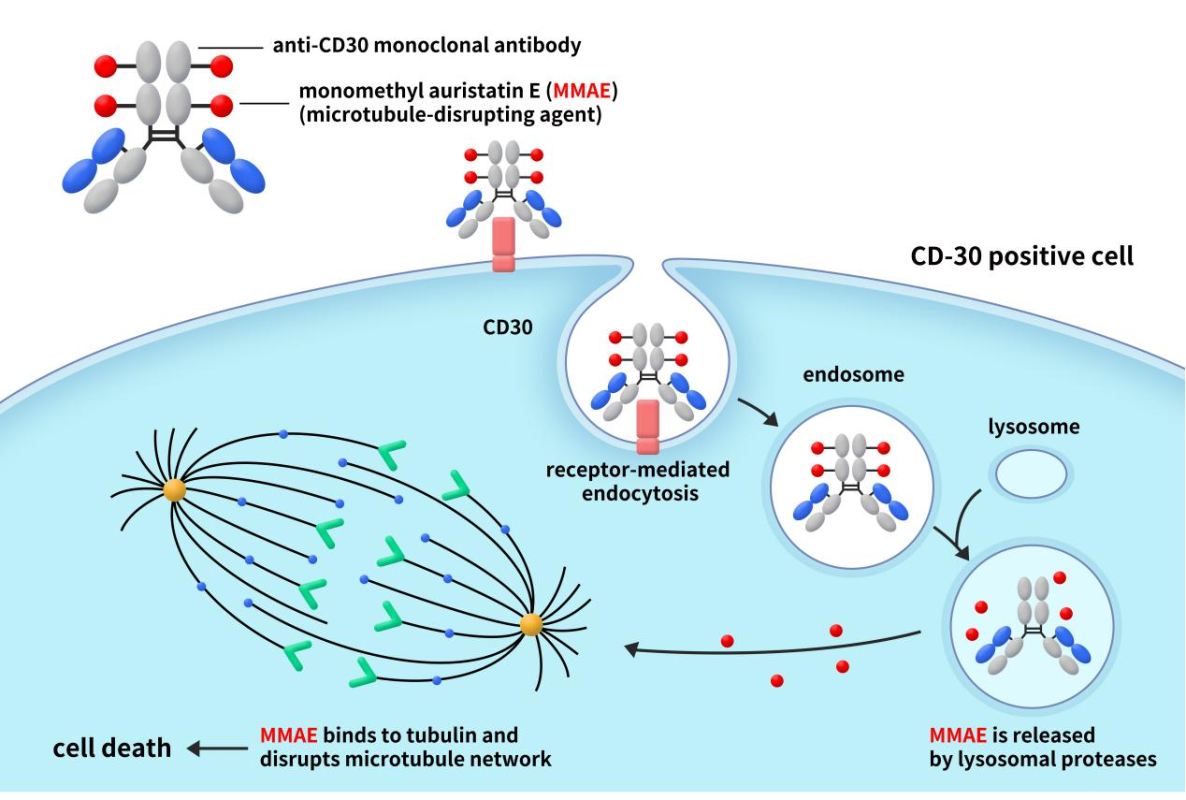
Figure 2. the mechanism of action of Adcetris [3]
As depicted in Figure 2, when the antibody component of Adcetris binds to CD30 on the surface of cancer cells, the CD30-Adcetris complex undergoes endocytosis and is transported to lysosomes within the cell. While the MMAE-conjugated to the antibody and connected by the linker remains stable extracellularly, it is released once the ADC binds to the target cancer cell and enters the cell. This release occurs due to proteolytic cleavage by intracellular enzymes, allowing free MMAE to diffuse further into the cell. Inside the cell, MMAE binds to microtubule proteins, inhibiting microtubule polymerization and disrupting the microtubule network. This disruption halts cell division and proliferation, ultimately leading to cell cycle arrest at G2/M and cell death [4]. Furthermore, MMAE can escape from CD30-positive lymphoma cells into the surrounding tumor microenvironment, generating bystander effects and enhancing its anti-tumor action. This advantage effectively kills tumor cells but can also cause toxicity to healthy cells. Therefore, comprehensive analysis and characterization of produced ADCs, as well as in vivo pharmacology studies, are essential to ensure the safety of these drugs.
4. How Anti-MMAE Antibodies Facilitate ADC Drug Development
Given the high cytotoxicity of ADC toxins, studying the pharmacokinetics of ADC payloads is crucial to understanding their behavior. However, due to MMAE’s inherent toxicity, in vivo studies in humans are not possible. Therefore, preclinical assessment of MMAE levels, such as determining ADC concentrations and conjugated MMAE concentrations, is essential. To address this, DIMA Biotech has developed anti-MMAE antibodies suitable for ADC ELISA assays. These antibodies enable the quantitative measurement of anti-MMAE-conjugates in test samples, aiding pharmacokinetic studies and DAR value analysis, thus expediting the development process of ADC drugs.
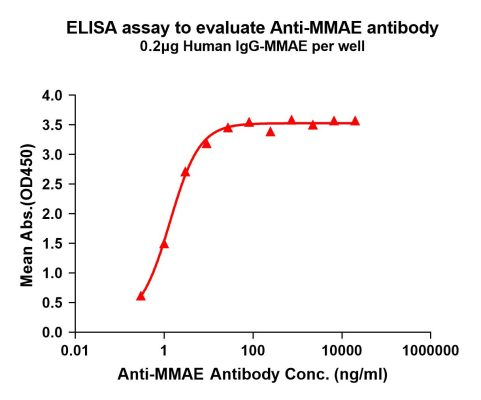
Figure 3. Elisa plates were pre-coated with IgG-MMAE (0.2μg/per well). Serial diluted anti-MMAE monoclonal antibody (DME101004) solutions were added, washed, and incubated with secondary antibody before Elisa reading. From above data, the EC50 for anti-MMAE monoclonal antibody binding with IgG-MMAE is 1.356ng/ml.
- More anti-MMAE mAb products
Cat No. | Product Name |
DME101007 | Anti-MMAE antibody(11C8); Rabbit mAb |
DME101006 | Anti-MMAE antibody(11B2); Rabbit mAb |
DME101005 | Anti-MMAE antibody(9C4); Rabbit mAb |
DME101003 | Anti-MMAE antibody(8B4); Rabbit mAb |
Reference:
[1] Wang Z, Li H, Gou L, Li W, Wang Y. Antibody-drug conjugates: Recent advances in payloads. Acta Pharm Sin B. 2023 Oct;13(10):4025-4059. doi: 10.1016/j.apsb.2023.06.015. Epub 2023 Jun 30.
[2] Dosio, F.; Brusa, P.; Cattel, L. Immunotoxins and Anticancer Drug Conjugate Assemblies: The Role of the Linkage between Components. Toxins 2011, 3, 848-883.
[3] Graham P Collins, David Bruce, Toby A Eyre. New Therapies in T-cell Lymphoma [J]. Lymphoma and Chronic Lymphocytic Leukemias. 2014, 1(4):1-8.
[4] Kovtun YV, Audette CA, Ye Y, Xie H, Ruberti MF, Phinney SJ, Leece BA, Chittenden T, Blättler WA, Goldmacher VS. Antibody-drug conjugates designed to eradicate tumors with homogeneous and heterogeneous expression of the target antigen. Cancer Res. 2006 Mar 15;66(6):3214-21.
[5] O’Connor O.A., Lue J.K., Sawas A., Amengual J.E., Deng C.C., Kalac M., Falchi L., Marchi E., Turenne I., Lichtenstein R., et al. Brentuximab vedotin plus bendamustine in relapsed or refractory Hodgkin’s lymphoma: an international, multicentre, single-arm, phase 1-2 trial. Lancet Oncol. 2018;19:257–266.