In 2012, a young girl named Emily Whitehead, suffering from acute lymphoblastic leukemia, became the first child to undergo second-generation CD19 CAR-T cell therapy by Dr. Carl June, a scientist in Pennsylvania. Her successful treatment marked a historic milestone in the world of medicine. It brought “CAR-T cell immunotherapy,” a revolutionary approach in the fight against cancer, to the limelight. The success of this therapy paved the way for a plethora of clinical applications. Since the FDA approved two CAR-T therapy products in 2017, the United States has witnessed the emergence of six CAR-T-related treatments. While China may have entered this field relatively later, it now boasts three CAR-T products on the market. As of now, the global CAR-T research pipeline is expanding rapidly, not only exploring new targets but also extending its applications to solid tumors. So, what exactly is CAR-T, and how is it positioned in hematologic malignancies and solid tumors? But before delving into CAR-T therapy, let’s take a step back and understand the evolution of CAR molecules.
- The Evolution of CAR Molecules
- How Does CAR-T Therapy Work?
- CAR-T Targets in Hematologic Malignancies and Solid Tumors
- DIMA’s CAR-T Lead Antibody Molecules Screening Platform
1. The Evolution of CAR Molecules
The concept of Chimeric Antigen Receptors (CARs) can be traced back to 1989 when Israeli scientist Prof. Zelig Eshhar discovered the structural resemblance between the antibodies produced by B cells and the T cell receptors (TCRs). Both had constant and variable regions. While antibody variable regions can recognize specific antigens, TCRs can only bind to antigen fragments presented by the Major Histocompatibility Complex (MHC). Eshhar proposed the idea of a “Chimeric Antigen Receptor” (CAR), which involved transferring the variable regions of antibodies to the constant regions of TCRs. This breakthrough opened up a new realm of possibilities for altering the antigen specificity of TCRs. Eshhar’s first-generation CARs comprised three parts: an extracellular domain for antigen binding, a transmembrane domain, and an intracellular domain for signal transduction.
Fast forward over 30 years, and CAR-T therapy has undergone multiple iterations, progressing from the first generation to the fourth generation, with each generation exhibiting unique characteristics.
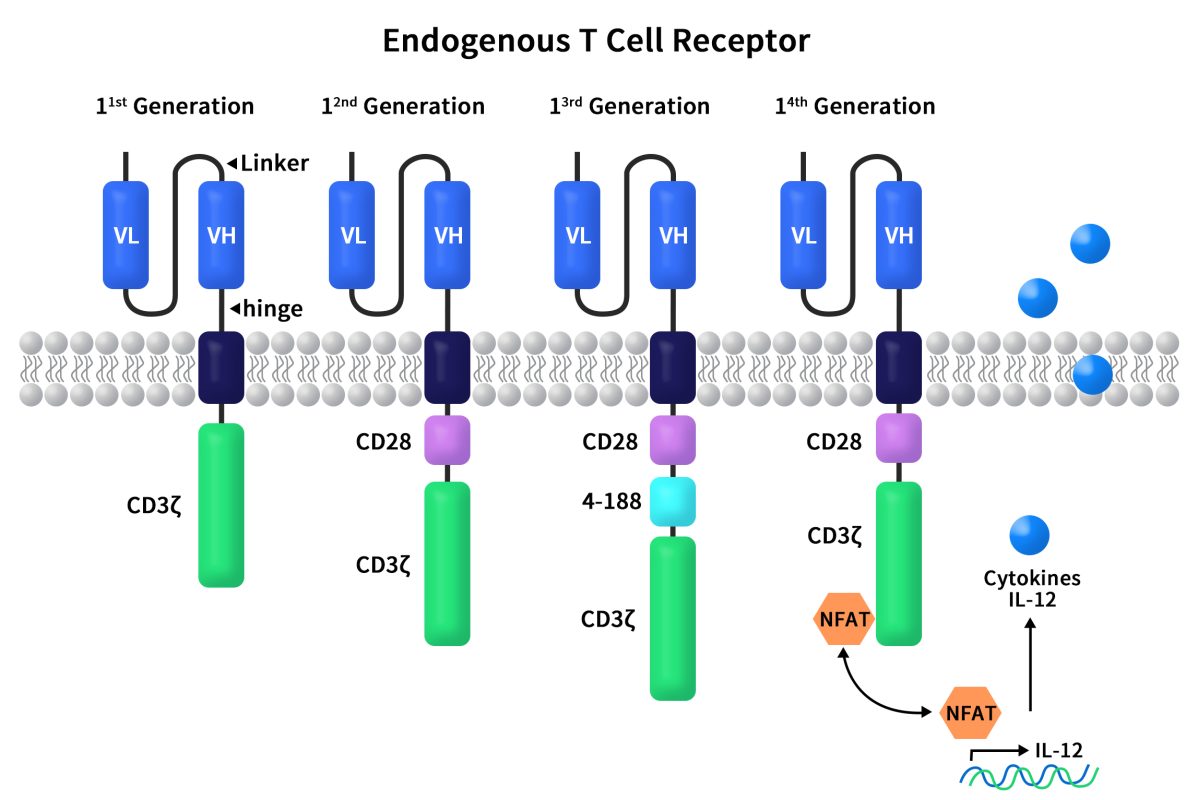
Figure 1. The structures of diverse CAR molecules
First-generation CARs, including CD3ζ and scFv, directly fused the scFv region of an antibody with CD3ζ. These T cells displayed anti-cancer activity in murine models. However, the first-generation CAR-T cells had slow expansion and poor persistence, leading to unsatisfactory results in clinical trials and cancer treatment.
Compared to first-generation CARs, second-generation CARs added an additional co-stimulatory receptor signal. This allowed CAR-T cells to accurately locate cancer cells while efficiently expanding and effectively killing tumors. The co-stimulatory receptor signal could originate from CD28 or 4-1BB, situated between the transmembrane region and the CD3 signal transduction domain. Second-generation CARs have the most clinical data, the highest stability, and the most mature processes.
Third-generation CARs introduced an additional co-stimulatory domain (CD28 and 4-1BB or OX40) onto the second generation to enhance signal transduction. However, the correlation between the number of co-stimulatory signals and signal transduction effectiveness is not always linear.
Fourth-generation CARs built upon the second-generation and introduced an intracellular domain that co-expressed certain small molecules like pro-inflammatory cytokine IL-12. This could trigger cytokine-induced signaling pathways or block some signaling pathways that affect CAR-T cell function. In preclinical models, CAR-T cell therapy efficacy was greatly enhanced by the presence of co-expressed cytokine structural domains compared to the second generation. This also successfully avoided systemic toxicity.
2. How Does CAR-T Therapy Work?
Chimeric Antigen Receptor T-cell (CAR-T) immunotherapy is designed to equip T cells with an entirely new targeting and activation function. When the antigen in question is found on the surface of cancer cells, T cells can specifically bind to and kill these cancer cells through cytotoxic action. In a clinical context, CAR-T therapy typically involves five steps:
Isolating T cells from the patient’s peripheral blood using leukapheresis.
Genetically engineering CAR-T cells in vitro.
Expanding and cultivating CAR-T cells in vitro.
Infusing a sufficient number of CAR-T cells back into the patient.
The CAR-T cell’s extracellular domain binds to the cancer cell’s surface antigen, leading to the cancer cell’s destruction. Close monitoring of the patient’s physical reactions is essential in the days following the infusion.
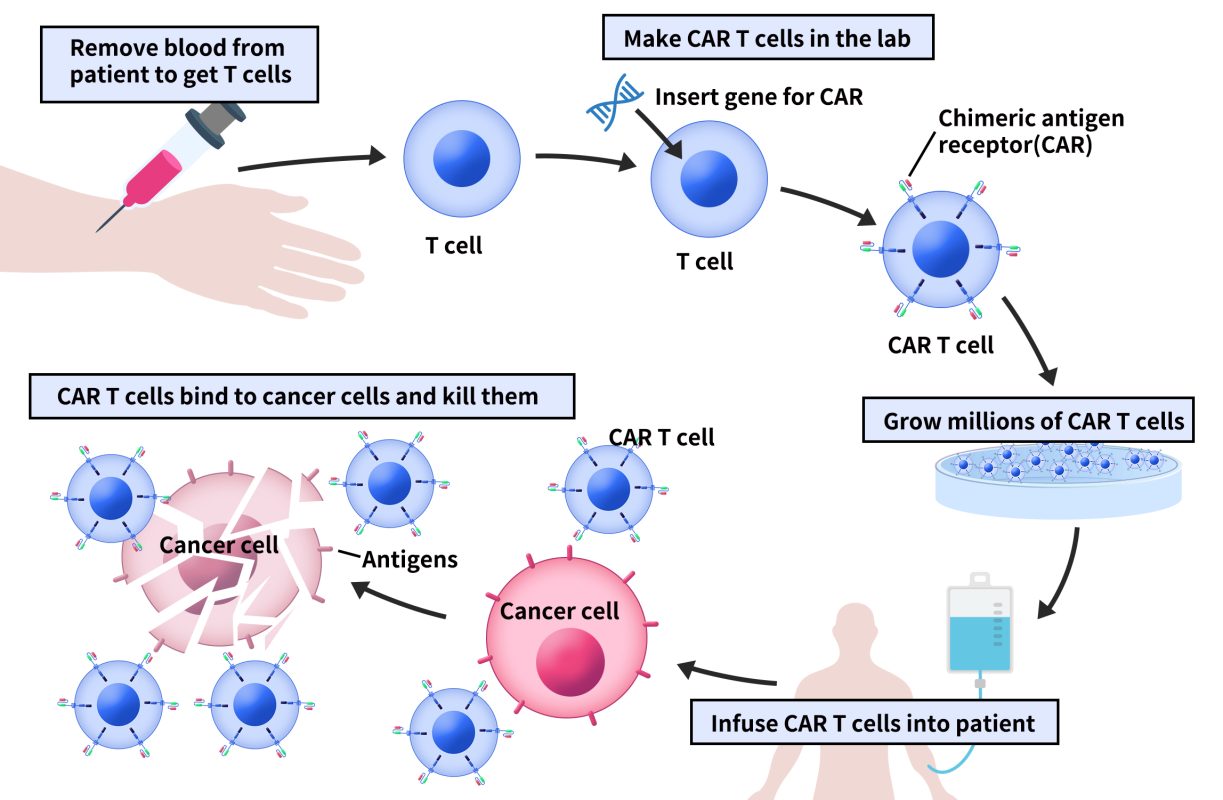
Figure 2. The process of CAR-T therapy
3. CAR-T Targets in Hematologic Malignancies and Solid Tumors
Since Emily’s successful CAR-T treatment in 2012, CAR-T therapy has achieved remarkable results in the realm of hematologic malignancies. It’s now hailed as one of the most promising cancer immunotherapies. As CAR-T therapy continually evolves and improves, its indications are gradually expanding into the treatment of solid tumors. However, whether for hematologic malignancies or solid tumors, the key to successful CAR-T therapy lies in selecting the right target. The choice of CAR-T targets is based on two primary factors:
The target should be specifically expressed on the cancer cell.
The target should be recognizable and attackable by CAR-T cells through effector molecules.
3.1 Popular CAR-T Targets in Hematologic Malignancies
As of now, eight CAR-T therapy products are approved worldwide for hematologic malignancies, with nearly 350 CAR-T products in clinical development. The major indications are focused on lymphocytic leukemia and non-Hodgkin’s lymphoma. While there are over 20 potential CAR-T targets in hematologic malignancies, CD19 and BCMA have shown the most clinical progress. Other notable targets include CD138, CD33, CD7, CD22, CD20, CD30, CD123, among others. Furthermore, ongoing research has introduced emerging targets like CD38, GPRC5D, and CD33.
CD19, an I-type single-pass transmembrane glycoprotein, is predominantly expressed during the early and mature stages of B cell development, making it one of the key biomarkers on the B cell surface. CD19, in combination with complement receptors CD21 and CD81, forms the primary signaling component in the mature B cell’s surface multimolecular complex. CD19 is the most frequently used CAR-T target in clinical projects related to hematologic malignancies. CAR-T products targeting CD19 include single-target CARs and dual-target CARs, often in combination with CD22 or CD20.
BCMA, a type III single-pass transmembrane protein, also known as TNFRSF17, belongs to the tumor necrosis factor receptor (TNFR) family. It’s mainly expressed on the surface of mature B lymphocytes and plasma cells, making it a significant marker for mature B lymphocytes. For multiple myeloma indications, BCMA is a primary target.
GPRC5D is a member of the G-protein-coupled receptor family, with seven transmembrane domains, and it’s specifically highly expressed in multiple myeloma cells. Eight projects with GPRC5D as the target are currently ongoing, with four in phase II and four in phase I clinical trials. Three of these CAR-T products combine GPRC5D with BCMA.
CD38, a type II single-pass transmembrane glycoprotein with ectoenzyme activity, is expressed at low levels in plasma cells and other lymphoid and myeloid cell populations. It’s a key target for multiple myeloma treatment
3.2 Popular CAR-T Targets in Solid Tumors
Unlike hematologic malignancies, CAR-T therapy for solid tumors had a later start. Currently, there are around 50 CAR-T products in clinical development for solid tumors, with most still in preclinical research phases. Prominent CAR-T targets for solid tumors include MSLN, Claudin 18.2, PD-1, HER2, NKG2D ligands, and CEACAM5. Ongoing research has introduced targets like ROR1, GD2, GPC3, CD70, MUC1, CLDN6, CTLA4, EGFR, AXL, and FAP. The indications for these CAR-T therapies mainly revolve around cancers such as esophageal, gastric, liver, colorectal, pancreatic, and biliary tract cancers.
MSLN (Mesothelin) is a cell surface glycoprotein extensively expressed in various tumors, including malignant mesothelioma, pancreatic cancer, and ovarian cancer. In solid tumor indications, there are ten CAR-T projects targeting MSLN. Four are in phase II clinical trials, while six are in phase I clinical trials, with an additional five projects in preclinical research.
Claudin 18.2, a member of the Claudin family of tight junction proteins, is a tetra-transmembrane protein. In normal tissues, Claudin 18.2 is exclusively expressed in differentiated gastric mucosal epithelial cells. However, in various tumors such as gastric and esophageal cancer, its expression significantly increases. Currently, ten projects involve Claudin 18.2 as the target for solid tumor indications. Four are in phase II clinical trials, six are in phase I clinical trials, and five are in preclinical research.
4. DIMA’s CAR-T Lead Antibody Molecules Screening Platform
As mentioned before, CAR-T therapy is to kill tumors by modifying the patient’s own T cells, making them more accessible to specifically bind to tumor cells. During the development of CAR-T therapy, early-stage CAR-T lead antibody molecular screening is an important basis for the clinical transformation of CAR-T products and is also the key to ensuring the clinical effectiveness and safety of CAR-T. DIMA is committed to the development of tumor immune target lead molecules and has successfully established a complete set of CAR-T lead molecule screening experimental platforms.
4.1 Workflow
Since the end of 2019, DIMA has accomplished the discovery of antibody lead molecules targeting more than 300drug targets, over 20 of which have been validated in vivo and in vitro CAR-T experiments, such as BCMA, CD123, CD38, CD70, GPRC5D, FCRL5, 5T4, CLDN18.2 and so on. Moreover, DIMA now has a good CAR-T cell preparation system, and customers can directly take CAR-T cells from us for killing and other functional experiments.
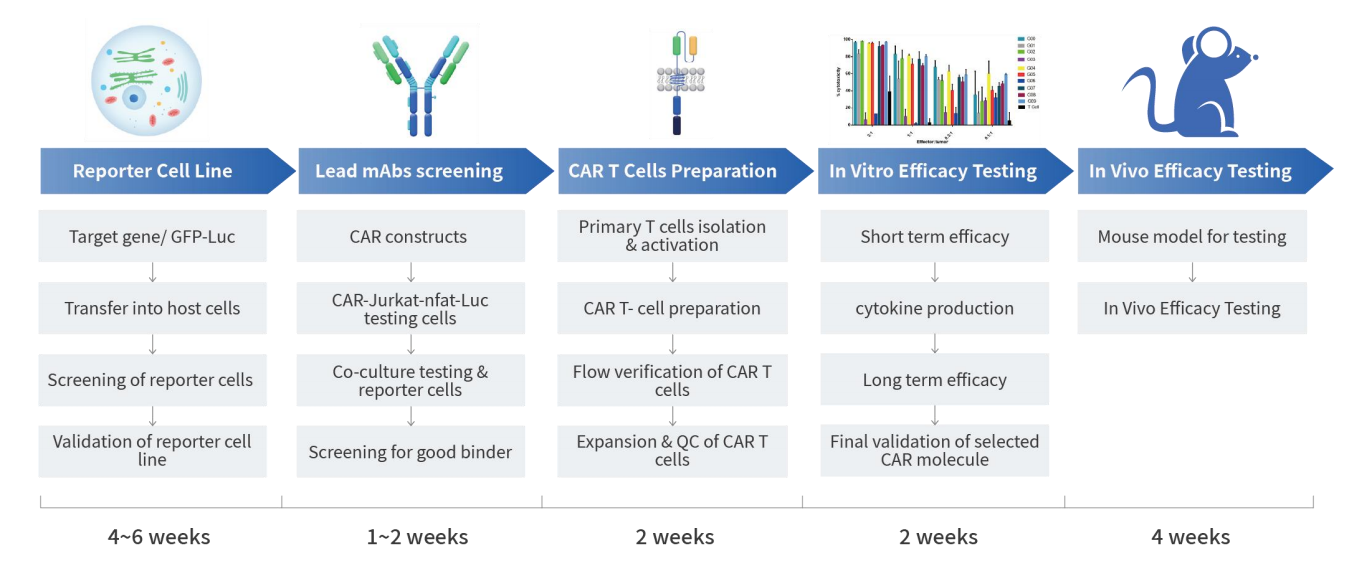
4.2 Partly in Vivo and in Vitro CAR-T Experiments Data
- Candidate antibodies were cross-tested with monkeys and mice
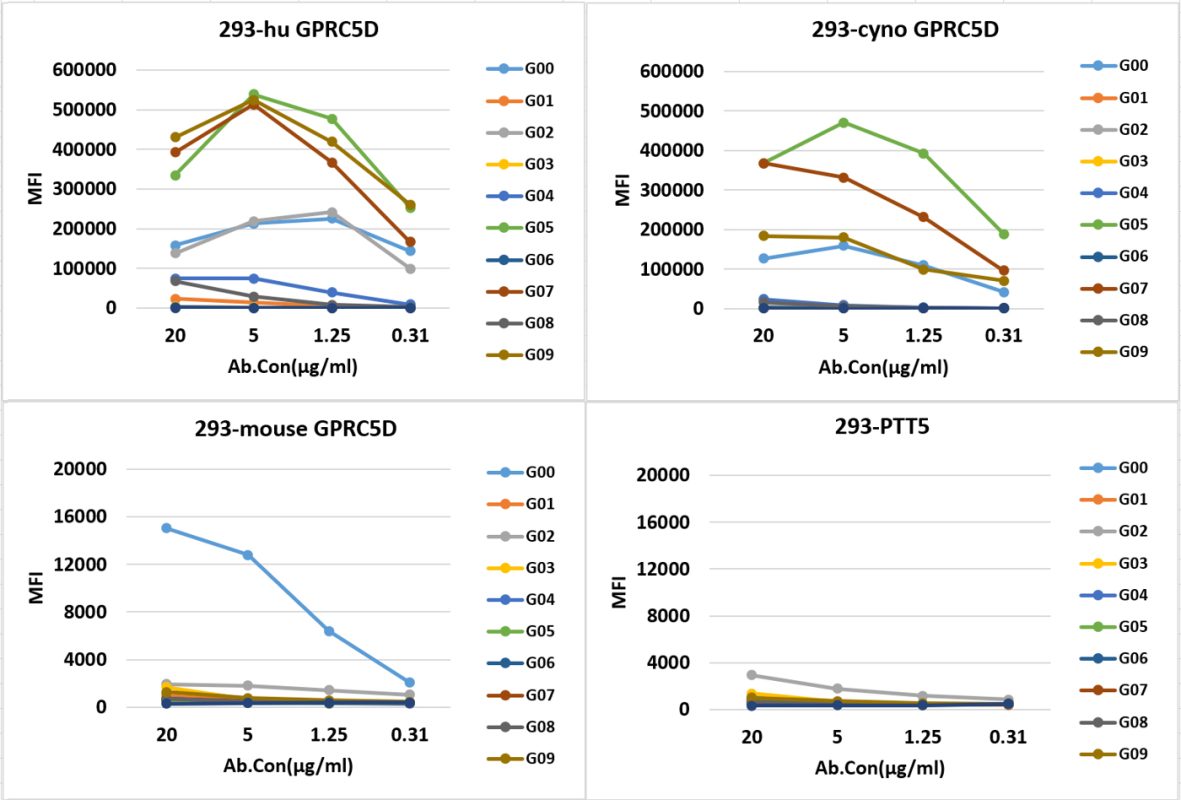
- CAR-T cells short-term killing tests were conducted along with secretion detection of cytokines (TNF-α, IFN-γ)
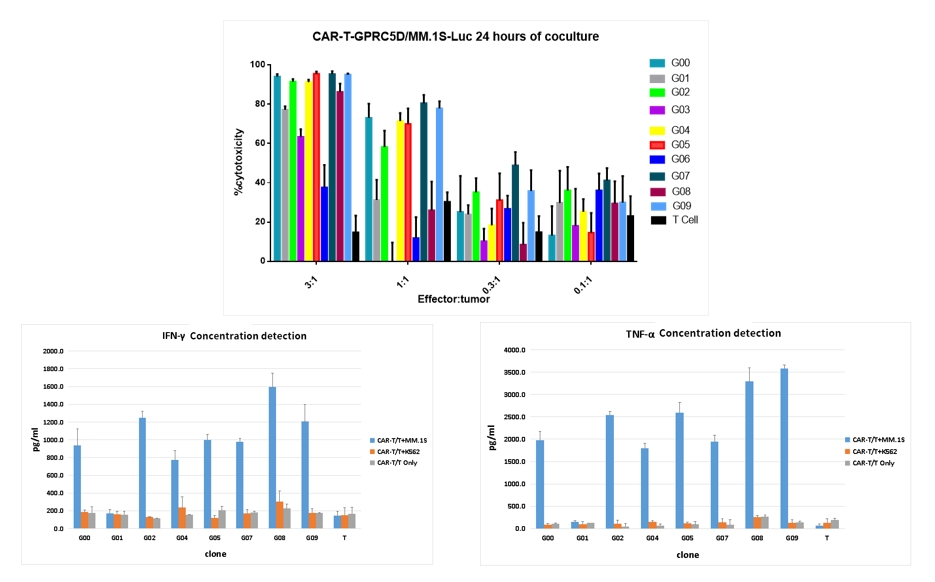
- CAR-T cell specificity activation and self-activation assessment
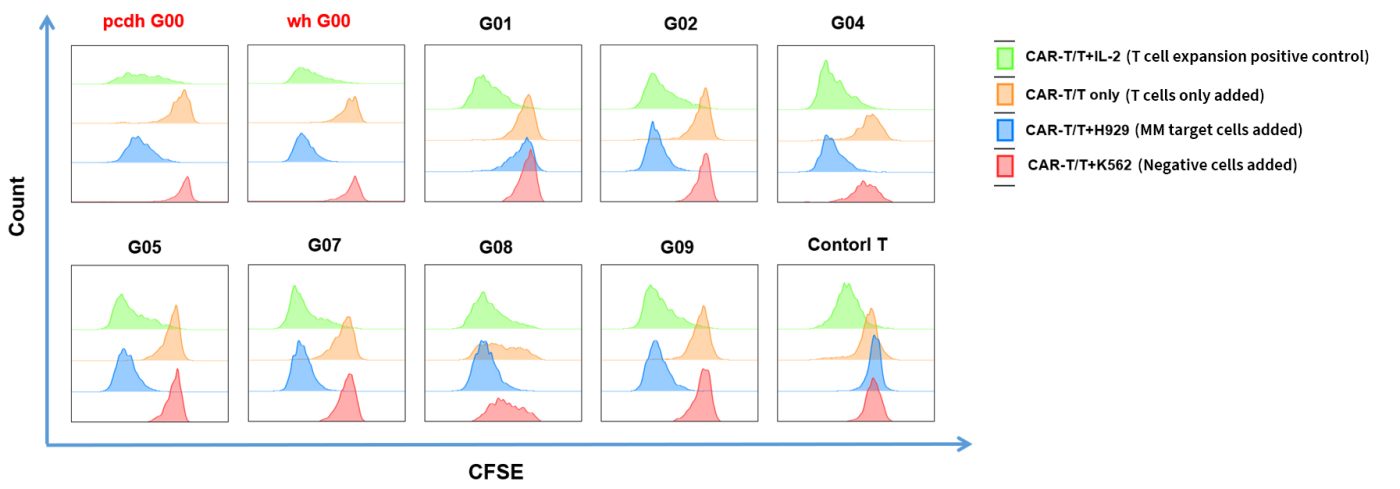
- Long-term cytotoxic function and proliferative capacity of CAR T cells
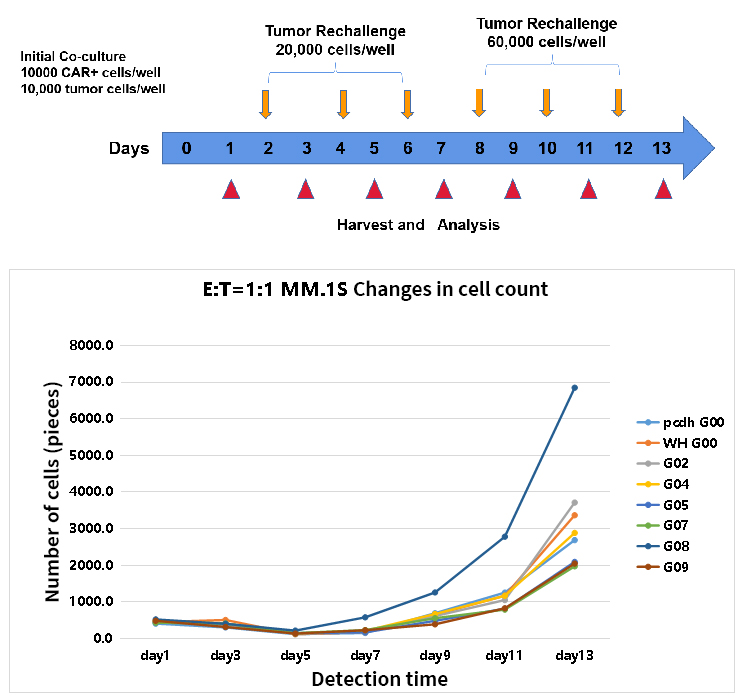
- Specific analysis of candidate molecules
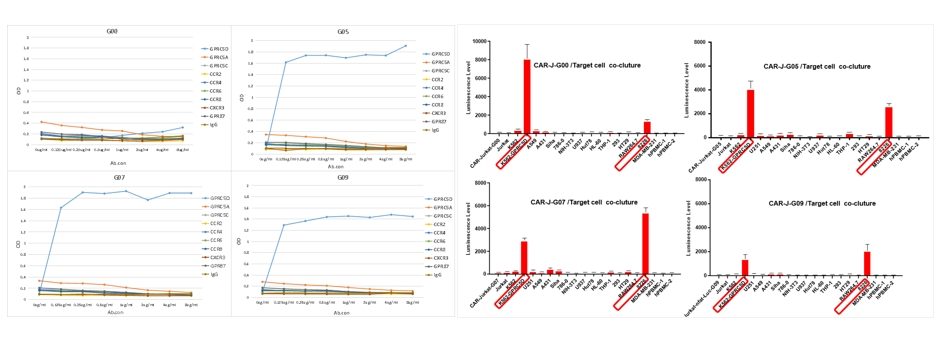
- In vivo animal pharmacology experiments for CAR-T cells
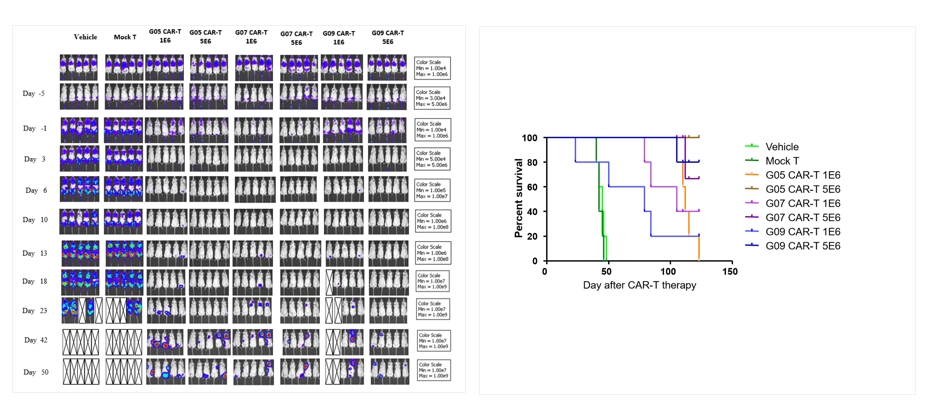
Through the settings of in vivo and in vitro experiments, we can screen whether the antibody part of the CAR molecule specifically targets cells, allowing you to better understand the characteristics of the target, the targeting selectivity, and the killing mechanism of the CAR molecule.
Chimeric Antigen Receptor T-cell (CAR-T) therapy represents a revolutionary approach to cancer treatment, as seen in the remarkable success stories of patients like Emily Whitehead. Over the past three decades, CAR-T technology has evolved significantly, with each generation enhancing its effectiveness and safety. CAR-T therapy offers new hope for patients with hematologic malignancies and is rapidly expanding its applications to solid tumors, ushering in a new era of cancer treatment. As research continues, the future of CAR-T therapy holds the promise of more advanced and effective treatments, bringing us closer to a world where cancer is no longer an insurmountable foe.
References:
[1] Mchayleh W, Bedi P, Sehgal R, Solh M. Chimeric Antigen Receptor T-Cells: The Future is Now. J Clin Med. 2019 Feb 7;8(2):207.[2] Hughes-Parry HE, Cross RS, Jenkins MR. The Evolving Protein Engineering in the Design of Chimeric Antigen Receptor T Cells. Int J Mol Sci. 2019 Dec 27;21(1):204.[3] Mitra A, Barua A, Huang L, Ganguly S, Feng Q, He B. From bench to bedside: the history and progress of CAR T cell therapy. Front Immunol. 2023 May 15;14:1188049.